Main Text
1 Introduction
Reproductive health is a basic component of a woman's physiological well-being, yet fertility is often taken for granted, and its complexities often overlooked. Infertility affects millions of women worldwide and is a condition that reproductive medicine has sought to resolve for decades. It is a complex issue that can be composed of a network of ailments within the reproductive tract, many of which are not understood. Infertility can be idiopathic, arising spontaneously, or iatrogenic, a result of a different medical treatment [1]. Further, environmental factors can affect the functionality of the reproductive tract [1]. Not only that, in our current modern western society women are choosing to have children later in life and they are faced with the realities of their reproductive timeline dictated by their ovaries. Therefore, it is important to be able to accurately assess a woman's ovarian fertility status and treat her accordingly. While infertility is extremely common, the various causes are yet to be fully understood. From idiopathic origins to cancer inducing infertility, Assisted Reproductive Technologies (ART) are often chosen during the course of treatment. According to the 2021 National ART Summary by the United States CDC, in the US 97, 128 live infants were born as a result of ART cycles began in 2021. Yet this option is costly, time consuming, and often not effective. Thus, further research is imperative in addressing infertility and advancing the field of medicine, and the nonhuman primate (NHP) model makes this possible.
The ovary is a highly studied reproductive organ as the producer of follicles and the source of the mature ovulated oocyte for fertilization. For this reason, the ovary plays an important role in infertility. A woman's reproductive potential is limited by her ovarian reserve, or the quantity and quality of oocytes that the ovary contains [2]. Reproductive aging is a natural and inevitable process resulting in a decline in the number of follicles present in the ovarian cortex throughout a woman's lifetime. However, it is also extremely variable amongst individuals, and a woman’s ovarian reserve is highly influenced by many factors such as genetic, mitochondrial, cytoplasmic, and environmental factors [3]. Historically, determining a person's ovarian reserve has been used as an indicator of their reproductive abilities and has been an important component to clinical decision making, especially in the realm of ART [4]. In fact, the ovarian reserve assessment is often more important than assessing a woman's age, due to it being one of the most important independent factors in female reproductive capability [5].
A woman's ovarian reserve is estimated based upon endocrine status evaluation and ultrasound ovarian assessment to determine antral follicle count [5]. Currently, follicle stimulating hormone (FSH) and anti-Mullerian hormone (AMH) are the two biochemical markers most often used clinically to measure ovarian reserve [3]. The two have complex interactions with each other as well as with the corresponding follicles of the ovary. AMH is currently known as a gonadal-specific glycoprotein in adult females that belongs to the transforming growth factor beta (TGF-β) superfamily [6]. AMH's physiological role in the ovary has been previously studied in mice; Salmon, Handyside, and Joyce confirmed that oocytes of primary and preantral follicles upregulated the expression of AMH mRNA levels in the granulosa cells of the ovary [7]. Another study done by Durlinger and colleagues determined that the secretion of AMH from growing follicles acts to inhibit primordial follicle initiation and growth [8]. Therefore, AMH serum levels of growing follicles are thought to regulate the primordial follicle pool, and therefore reflect the ovarian reserve.
Understanding the role of AMH in the ovary revealed the potential clinical value of AMH as a biochemical marker of a woman’s ovarian status, with value in predicting future fertility outcomes in women. In women with various degrees of premature ovarian failure, AMH has been validated as a reliable marker of the extent of follicular pool depletion and predictor of ovarian senescence [9,10]. Additionally, AMH is particularly relevant in determining ART fertility outcomes. Nelson et al. reported that AMH levels were determined to be an accurate and reliable predictor of ovarian response and thus live birth outcomes, in those undergoing ART treatment, and AMH had a higher predictive value than antral follicle count [11]. The importance of AMH in subjects with no known reproductive disorders has also been investigated. In a study involving bovine models, Ribeiro and associates reported a positive association between AMH and fertility in dairy cows; cows with low plasma AMH concentration had lower pregnancy rates and greater incidence of pregnancy loss [12]. Additionally, cows that failed to become pregnant by the end of the breeding season had a lower concentration of AMH in plasma than cows that became pregnant [12]. However, the reliability of AMH serum levels and its predictive ability are still in question, and its direct correlation to pregnancy outcomes needs further investigation. In a study that looked at AMH levels in women attempting to conceive naturally with no history of infertility, low AMH levels were not associated with a lower probability of conception [13]. Thus, while AMH’s physiological role in the ovary is relatively well understood, how it can effectively be used needs to be carefully considered.
As previously noted, reproductive function and follicle development is largely regulated by the complicated interactions of intraovarian hormones. While significant research has been conducted around the physiological role of AMH, reproductive biology still lacks a complete understanding of all ovarian hormones and their various roles in reproduction. Of specific interest is activin A, an ovarian hormone produced by the granulosa cells of primary and preantral follicles. Previous research has illustrated the importance of activins in folliculogenesis and reproductive biology [14]. Activins are a subfamily of the superfamily TGF-β and have a unique role regulating FSH, a highly important hormone in folliculogenesis [15], although this protein can also be found during gestation in primates, produced by the placenta. Activin receptor type II is the major activin receptor on the anterior pituitary [15]. A study that knocked out this receptor in mice caused a defect later in life, with few antral follicles and a higher incidence of follicular atresia, elucidating the potential impact of the presence of activins in the ovary [15]. A different study that modeled early folliculogenesis through an in vitro organ culture system in mice, established activin A's anti-apoptotic properties; primary and preantral follicles that were treated with activin A alone had an increase in healthy versus atretic follicles [16]. Therefore, activin A is thought to play an important role in early follicle development; however, the use of this hormone as a predictive measure of ovarian status has not been characterized. The relationship between activin A levels and the quality and quantity of follicles is unclear and needs further investigation in order to be able to incorporate this hormone into a clinical assessment of a woman's ovarian status.
The NHP model is an ideal candidate for reproductive research because of its direct translation and relevance to the human model. The rhesus macaque (Macaca mulatta), an Old World monkey originating in Asia, is a NHP species that has been used in biomedical research for decades across multiple disciplines, due to its phylogenetic proximity and genetic similarities to humans [17]. Furthermore, the female NHPs and women shares striking similarities in reproductive biology, from hormonal and neural control to local control of reproduction, offering research insights that other laboratory or agricultural models cannot [18]. Firstly, NHPs are unique in that they have a cyclical menstrual cycle similar to that of humans, dictated by the hormonal control of estrogen and progesterone, with subsequent uterine proliferation, differentiation, and sloughing [18]. The ovarian cycle is also similar in that ovarian follicle maturation is under the control of FSH and LH, resulting in follicular atresia or ovulation. This is followed by the formation of the corpus luteum, which lasts fourteen to sixteen days, until luteal cell apoptosis is complete [19]. NHPs have also been characterized as experiencing reproductive senescence and undergoing ovarian and neuroendocrine changes in the same way that humans do, resulting in a loss of menstruation later in life [20]. Further comparisons involve pregnancy and placentation in NHPs. NHPs display a hemochorial placenta known to be villous in structure within cotyledons, resulting in a direct contact of maternal blood with fetal tissue, comparable to humans [18]. Thus, these similarities allow novel findings that can be directly translated to humans. The NHP model provides opportunities that other animal models cannot, and has been utilized to accelerate the understanding of human reproductive biology, reiterating the continued importance today.
Based upon previous work and knowledge of AMH and activin A, this study sought to determine the relationship between hormone levels and establishment of early pregnancy. The objective of this study was to use the NHP model as a well-characterized animal model to assess the predictive power of AMH and activin A in naturally cycling individuals, and their impact on the resulting pregnancy status. We hypothesized that AMH and activin A measurements taken early in the ovulation cycle can be used to predict establishment of pregnancy in naturally cycling rhesus macaques, and therefore, there will be higher levels of AMH and activin A in the animals that became pregnant compared to those that did not. The reproductive cycles and follicle characteristics of NHPs are highly understood and extremely relevant to the human model, making this study not only relevant to future primate research, but also for clinical application in humans. A greater understanding of how to best utilize these ovarian hormone levels in NHPs may improve future research projects, offering a screening of candidates before beginning costly protocols. Additionally, this study could improve clinical decision making, screening, and treatment options for women seeking fertility consultation by offering a more accurate snapshot of their ovarian status and further cutting down potential costs and time spent for treatment.
2 Materials and methods
2.1 Animals
All animal procedures were approved by the Oregon Health & Science University (OHSU), Oregon National Primate Research Center (ONPRC) Institutional Animal Care and Use Committee (IACUC) and comply with the Animal Welfare Act and the Guide for the Care and Use of Laboratory Animals (The Guide; https://doi.org/10.17226/12910).
Forty-seven female rhesus macaques ( Macaca mulatta) from the Rhesus Macaque SPF4 breeding colony were primarily housed outdoors in seven one-acre corrals, sheltered housing units, and indoor/outdoor corn cribs at OHSU's ONPRC, Beaverton, OR and maintained by the ONPRC Division of Comparative Medicine (DCM). The female macaques were all naturally cycling animals with no known reproductive health issues. The female NHPs were of various ages, ranging from 6.3 to 17.7 years old, with an average age of 13 years old. The female NHPs were of various weights, ranging from 5.35-10 kg, with an average weight of 7.35 kg. All animals are weighed on a monthly basis in the colony, and the last weight before onset of mating was used for evaluation. The study was performed as a retrospective, non-randomized, cohort analysis and was a population-based study in a colony of managed rhesus monkeys at a National Primate Center. These were reproductive aged females selected from the ONPRC colony as part of the Timed-Mated Breeding (TMB) Resource run in collaboration between the ONPRC DCM and the ONPRC ART Core [21]. Samples were obtained during the breeding season until females either became pregnant, or were released from the program (as described below). In all, data from 90 menstrual cycles were collected from this cohort.
Behavioral management of all NHPs is overseen by the Behavioral Services Unit (BSU) at ONPRC, including the training and conditioning of the animals for awake blood sample collections, reducing stress associated with the event. The animals were monitored by DCM staff daily for the detection of menses. Onset of menses were determined by visual inspection of perianal area daily, and in some females with daily vaginal swabbing for presence of menstrual bleeding, similar to previous studies in rhesus macaques within this colony [22]. After menses had been detected, daily blood collections began approximately 7 days post-menses using a bleed box to secure the animal and allow for a safe collection. 1 mL of blood was collected, beginning at around day 7 post-menses; however, this varied from day 5 post-menses to day 15 post-menses, depending on the animal's menstrual cycle history and were timed to allow for detection of the rise of estradiol (E2), indicating pending ovulation. Daily blood draws continued until ovulation was imminent. The whole blood samples were centrifuged (1,000 x g for 15 minutes at 4 ℃) to retrieve serum. Sera were assayed by the Endocrine Service Core at ONPRC, to measure E2 and progesterone (P4) by use of an automated radioimmunoassay platform validated for NHP serum [23]. E2 and P4 levels were utilized as an indicator of ovulation and luteinization in the female NHP, indicating a cycle had a high probability of fertility. When daily E2 > 100 pg/mL, indicating ovulation was likely, the female was then pair-housed with a fertile male monkey in a TMB protocol (Figure 1). Blood collections continued in order to monitor a rapid surge and then decline in E2 serum levels with P4 levels on the rise. As P4 levels approached 1.0 ng/mL, the animals were unpaired from one another. Daily bleeds were discontinued. After 2.5 and 3.5 weeks post pairing, 1 mL of blood was collected and submitted to the Endocrine Service Core to measure P4 levels as an indicator of pregnancy. Pregnancy status was determined via ultrasound around embryo day 30, for visualization of gestational sac [22]. Females were re-paired with males if they failed to become pregnant until the end of the breeding season. Serum samples were placed in either a -20 ℃ freezer or -80 ℃ freezer to be safely stored and used later for analysis.
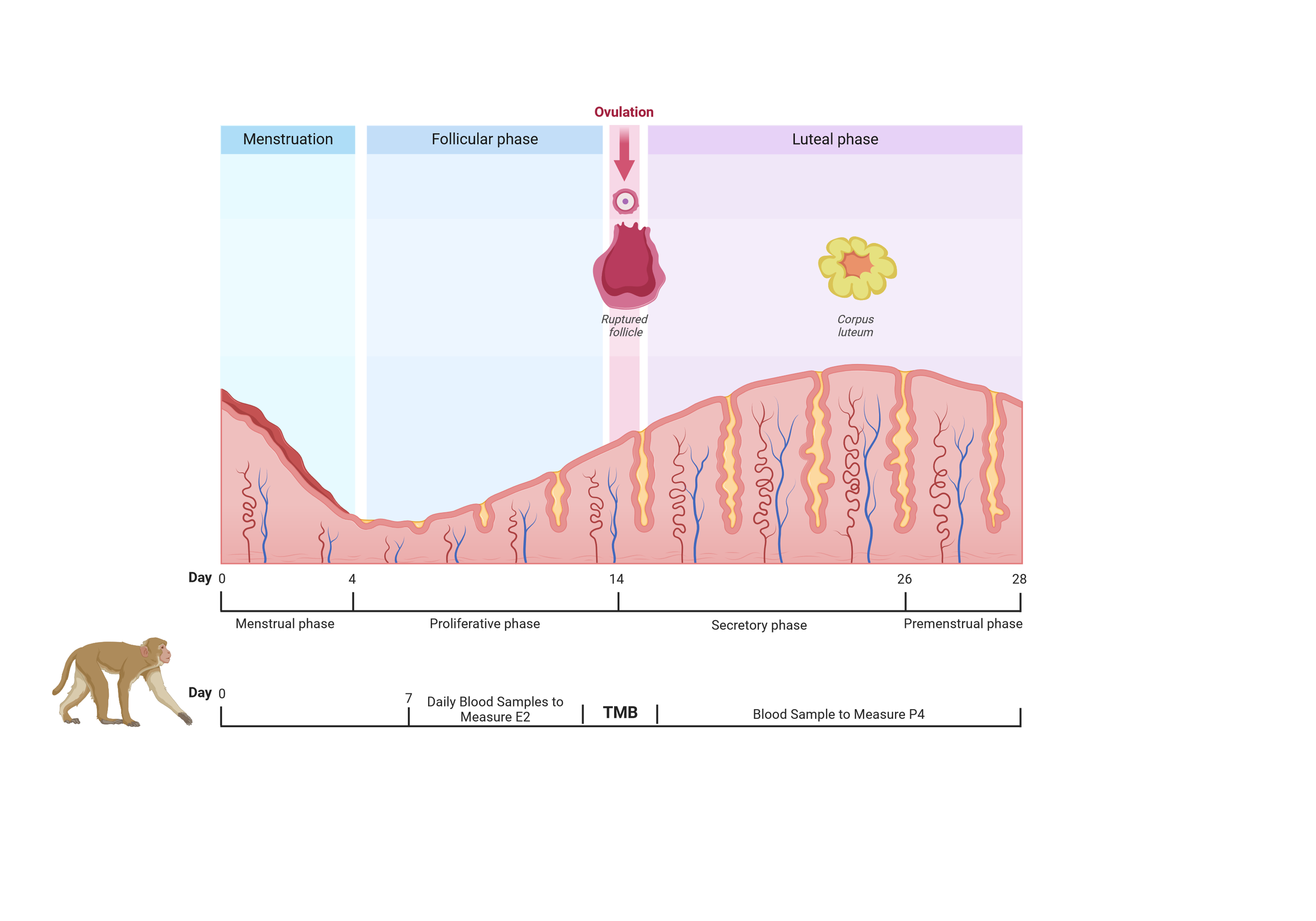
Figure 1 Summary of timed-mated breeding (TMB) timeline in conjunction with the hormonal monitoring of the animals at the corresponding time in the ovarian cycle. Daily blood sample collections began around day 7 post menses to measure estradiol (E2) and progesterone (P4) and predict time of ovulation. At the time of ovulation, a female was paired with a male (TMB), followed by the continued measuring of P4 to determine unpairing time and pregnancy. (Created with https://www.biorender.com/).
2.2 Hormone Analyses
The first sample taken from the daily blood draws was utilized for analysis of serum levels of AMH and activin A in each TMB cycle. Enzyme linked immunosorbent assays (ELISA) that were previously validated in NHPs were used to measure both AMH and activin A [24]. The Ultra-Sensitive AMH/MIS ELISA RUO AL-110 (Ansh Labs, Webster, TX, USA) kit was used to measure AMH and the Activin A ELISA AL-110 (Ansh Labs, Webster, TX, USA) kit was used to measure activin A. All assays were conducted by a single person that was blinded to all concentrations of other hormones and pregnancy outcomes. The researcher only knew the animal ID and sample date of the serum collected while conducting the assays.
All serum samples to be used were thawed on ice before beginning the ELISA procedure. In the instance that a large clot was detected in the samples that would interfere with retrieval of the serum, the samples were centrifuged for pure serum retrieval (8,000 x g, Legend Micro 21R, for 1 minute at 4 ℃). Assays were completed to quantify AMH and Activin A concentrations as suggested by manufacturer's protocols (Ansh Labs, Webster, TX). Following the completion of both ELISA procedures, the optical density absorbance wavelength of each well was determined immediately using a Versamax Tunable Microplate Reader (Molecular Devices, LLC, San Jose, CA, USA) at 450 nm, and data analyzed using OriginPro 2019 (OriginLab Corporation, Northampton, MA, USA). Using the known concentrations of the calibrators plotted against the corresponding optical densities, a standard curve was generated using a cubic regression curve fit function. The unknown concentrations of the hormone of interest were then extrapolated from the optical density data. The lower limit of sensitivity for AMH was 0.023 ng/mL. The resulting intra-assay coefficient of variation of all AMH/MIS ELISAs completed was 7.9%, while the inter-assay coefficient of variation was 8.9. The lower limit of sensitivity for activin A was 0.065 ng/mL. The resulting intra-assay coefficient of variation for all activin A ELISAs completed was 5.97%, while the inter-assay coefficient of variation was 7.3%.
2.3 Statistical Analyses
The concentrations of AMH and activin A were analyzed using linear models function of SAS (SAS Enterprise Guide version 8.2; Cary, NC, USA) including AMH by pregnancy status and activin A by pregnancy status, as well as age and weight by pregnancy status. Additionally, the Linear Regression Analysis of SAS with Spearman Bias Adjustment for multiple correlations was used to detect correlations between AMH vs E2, AMH vs age, and AMH vs body weight; activin A vs E2, activin A vs age and activin A vs body weight. A sub-analysis of each correlation within all females who became pregnant and all females who failed to become pregnant was used. Data were considered statistically significant if p < 0.05, and trends were noted for p = 0.051-0.10. A linear models sub analysis was conducted including only younger females (15 and younger), as rhesus females >15 years are considered nearing the end of their reproductive lifespan and follicle cohort declines significantly above 15 years of age [25] and chances of successful pregnancy declines above 15 years of age [26].
3 Results
Of the 90 samples that were collected from 47 females, 62 cycles did not result in a pregnancy, while 28 cycles did result in a pregnancy (Figure S1). There was variation in the day of the cycle in which the sample was collected, as there was variation in ovulation times depending on the individual. Over half of the samples were collected on day 7 after menses; however, samples from days 5-11 post-menses, as well as day 15 post-menses were also included (Figure S2). As expected, there were no effects of body weight, nor age on pregnancy success rates (Figures S3 and S4), as all females were at a healthy body weight and had evidence of regular menstrual cycles which were criteria for inclusion in a rhesus breeding colony.
There was no significant difference in serum AMH by pregnancy status for all monkeys (p = 0.46; Figure 2). Analysis of only young monkeys (15 years and younger) also showed no significant difference of AMH by pregnancy status (p = 0.25; Figure 3). Similarly, there was no significant impact of activin A on pregnancy status for all monkeys; however, both groups (pregnant and nonpregnant cycles) had extreme outliers present (p = 0.3836; Figure 4). The age and weight of the monkeys were of importance to us in this study and taken into consideration. The impact of these additional factors on levels of AMH and activin A was evaluated. Linear Regression analyses revealed a significant negative correlation between age and AMH (R = -0.32; p = 0.002; Figure 5). When analyzed by TMB results for that cycle (pregnant cycles vs non-pregnant cycles), a similar negative correlation between age and AMH was detected when sample data from the fewer pregnant cycles analyzed were removed (Figure S5). There was also a significant negative correlation between weight and AMH with a 95% confidence interval (R = -0.22; p = 0.036; Figure 6 and Figure S5). There was a significant negative correlation between activin A and weight for only pregnant cycles, however, this data also included two extreme outliers (R = -0.38; p = 0.047; Figure 7 and Figure S6). Finally, no significant correlations were detected between serum E2 and AMH (R = -0.06; p = 0.56) or between E2 and activin A (R = -0.07; p = 0.54).
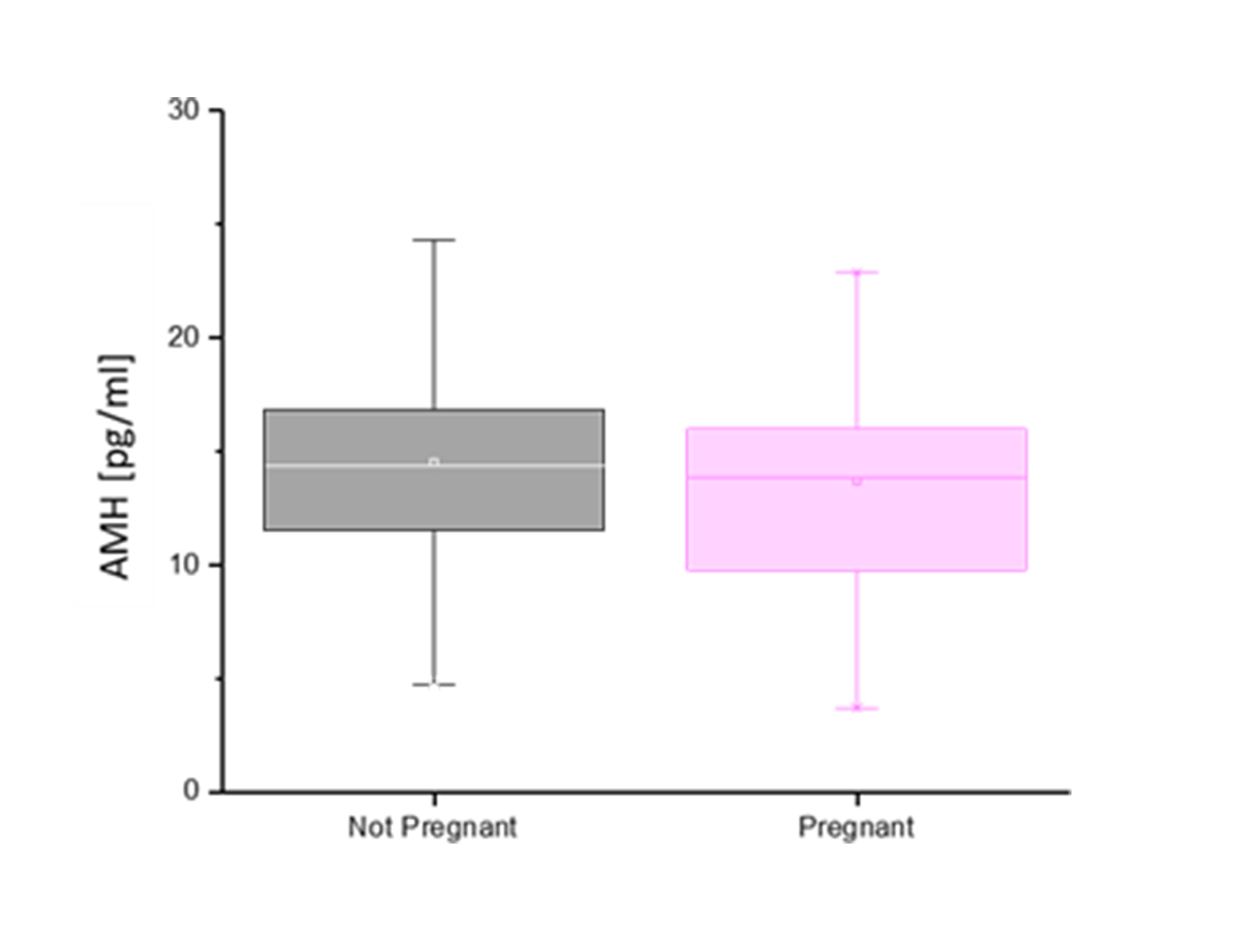
Figure 2 Anti Mullerian hormone concentrations (pg/mL) (y-axis) of initial blood sample collected relative to the pregnancy outcome (x-axis). No significant difference in AMH concentration based on pregnant (pink) versus non pregnant (gray) outcome, p = 0.46.
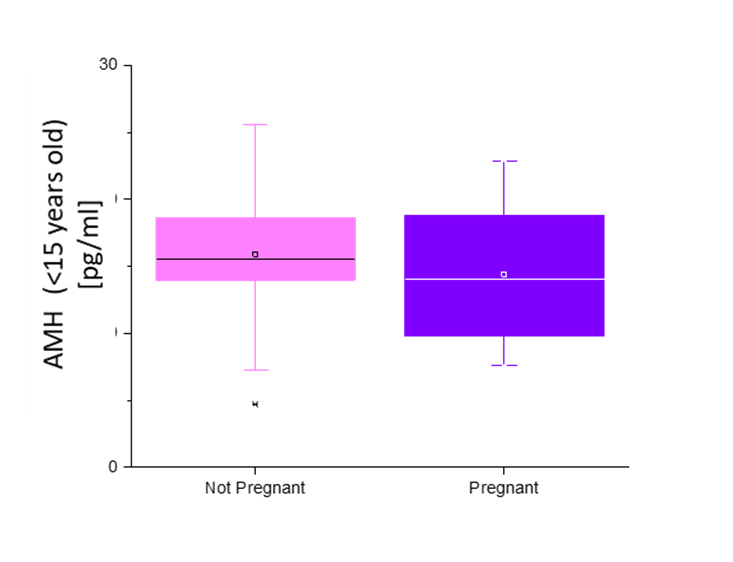
Figure 3 Anti Mullerian Hormone concentrations (pg/mL) of females ages fifteen and younger (y-axis) of initial blood sample collected relative to the pregnancy outcome (x-axis). No significant difference in AMH concentration based on pregnant (purple) versus non pregnant (pink) outcome, p = 0.25.
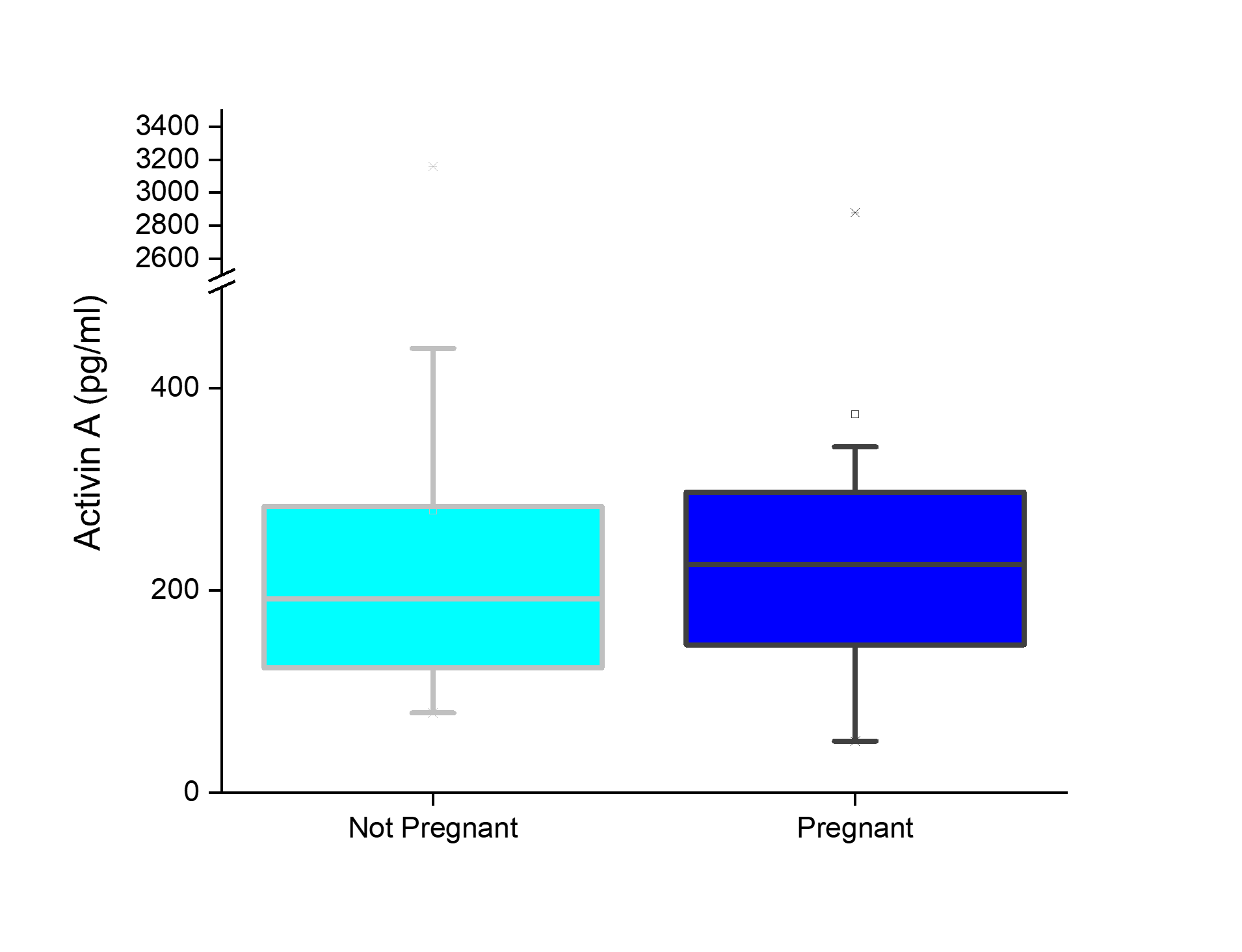
Figure 4 Activin A concentrations (pg/mL) (y-axis) of initial blood sample collected relative to the pregnancy outcome (x-axis). No significant difference in activin A concentration based on pregnant (dark blue) versus non pregnant (light blue) outcome, p = 0.38.
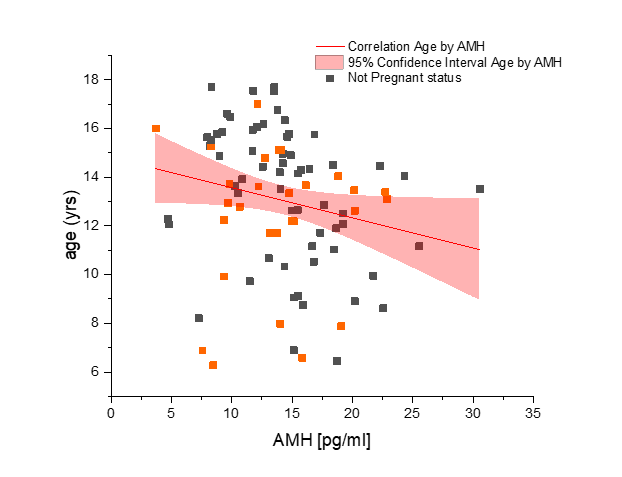
Figure 5 Evaluation of anti-Mullerian hormone concentration (AMH) (x-axis) and correlation to age of rhesus macaque females used in study (n = 47) (y-axis). Not pregnant females are represented in dark gray squares and pregnant females are depicted by orange squares. As a clarification, these were pre-mating samples from both groups combined. The linear correlation is shown by the red line. Significant correlation exists (p = 0.002) between AMH and age with 95% confidence, as seen within the light red region on the graph. Correlation with Spearman bias adjustment gives a negative slope of -0.32.
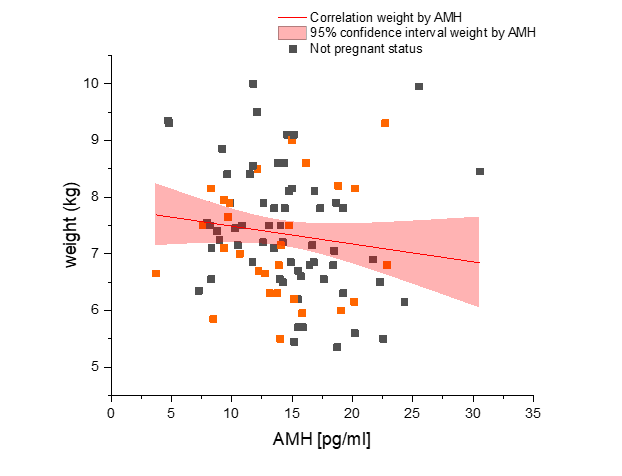
Figure 6 Evaluation of anti-Mullerian hormone concentration (AMH) (x-axis) and correlation to weight of rhesus macaque females used in study (n = 47) (y-axis). Not pregnant females are represented in dark gray squares and pregnant females are depicted by orange squares. As a clarification, these were pre-mating samples from both groups combined. The linear correlation is shown by the red line. Significant correlation exists (p = 0.036) between AMH and weight with 95% confidence, as seen within the light red region on the graph. Correlation with Spearman bias adjustment gives a negative slope of -0.22.
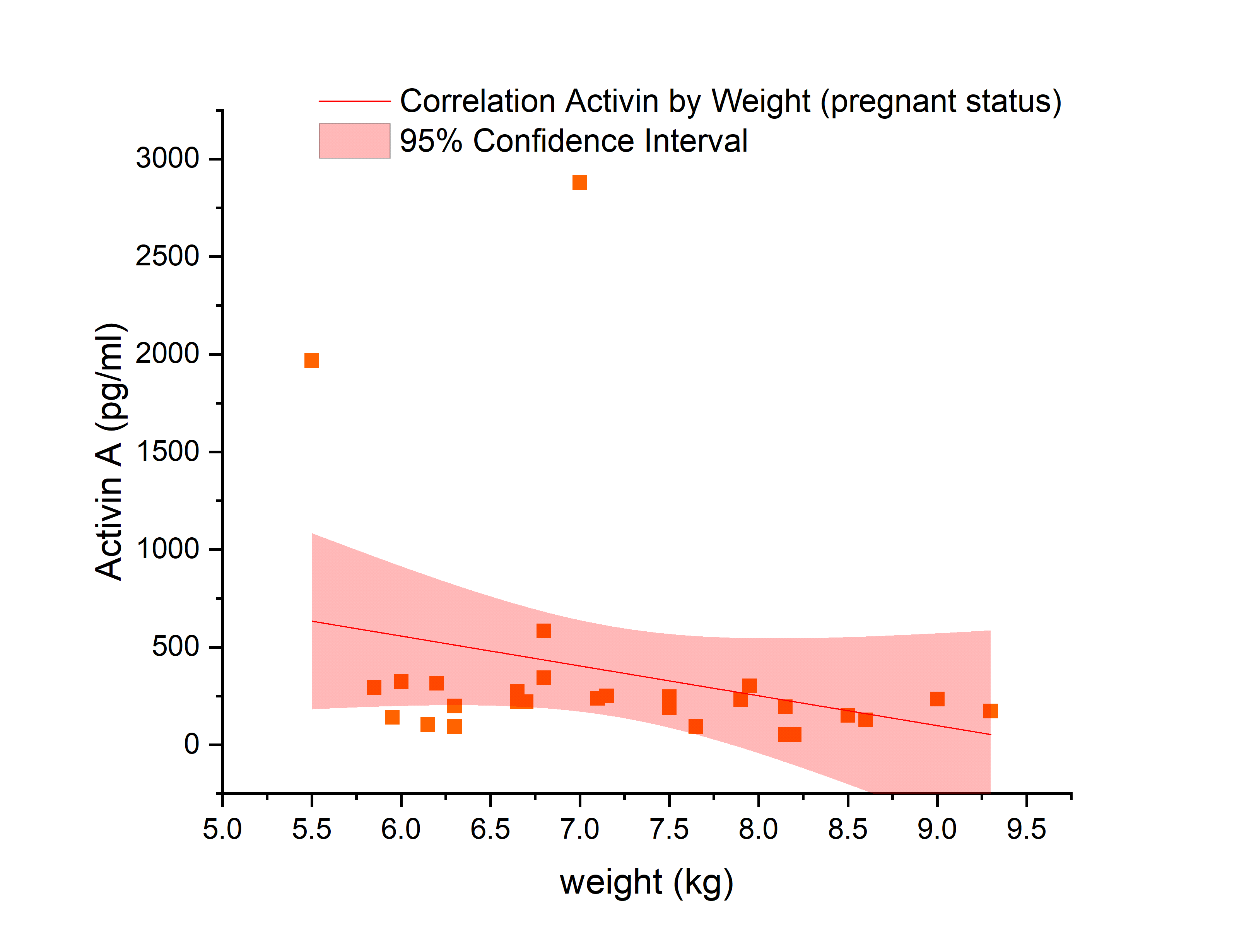
Figure 7 Evaluation of activin A concentration (pg/mL) (x-axis) and correlation to weight of rhesus macaque females used in study (n = 47) (y-axis) for only cycles that resulted in pregnancy. Pregnant females are depicted by orange squares. The linear correlation is shown by the red line. Significant correlation exists (p = 0.047) between activin A and weight in pregnant females only with 95% confidence, as seen within the light red region on the graph. Correlation with Spearman bias adjustment gives a negative slope of -0.38.
4 Discussion
Medicine has evolved to alleviate the burdens of infertility and ovarian aging through ART; however, with this costly and time-consuming process, successful pregnancy rates have been less than 50% in most fertile women over the last decade. Before proceeding with ART, prediction models are often created to assess various components of a women's reproductive history, including ovarian hormone levels, specifically AMH [27]. However, the predictive abilities and accuracy of AMH and other ovarian hormones is still unclear in fertile women. The direct impact of these hormones on pregnancy outcomes has yet to be characterized. A greater understanding of AMH and activin A and their role in fertility will facilitate more efficient and effective reproductive decisions.
In this study, we measured AMH and activin A levels in the serum of naturally cycling NHPs of various ages with no known reproductive health issues. We identified that neither AMH nor activin A levels are significantly predictive for establishment of early pregnancy. Both hormones are naturally occurring ovarian hormones secreted early during folliculogenesis. AMH has been previously characterized to reflect the number of growing follicles present in the ovary and is considered the best available measure of the ovarian reserve; a poor ovarian reserve is associated with reduced pregnancy rates [28]. Yet, previous studies consistent with our findings have found no difference in the overall follicular production of AMH in infertile patients compared to the fertile control group [29], and neither higher nor lower serum AMH levels were associated with fecundability [30]. Additionally, a study similar to this study examined the effects of AMH on natural pregnancy, suggesting a weak predictive value for AMH, and stating that decreased AMH values do not represent decreased natural fertility [31]. Yet, there may be an important distinction between the utilization of AMH for ART treatments compared to natural fertility decisions. In a study measuring low AMH levels due to severe endometriosis and the resulting oocyte yield, embryo quality, and pregnancy outcomes during IVF, there was no reduction in embryo quality or pregnancy outcomes, however, there was a reduction in oocyte yield [32]. This could show the value of AMH to predict the quantity of eggs retrieved if relevant to the ART treatment desired. Furthermore, previous studies in women report high follicular fluid AMH levels associated with successful oocyte fertilization in IVF cycles [33], as well as greater follicular growth and ovarian response to IVF treatment in patients with a higher AMH at initial screening [34].
Activin A has been previously characterized as having a unique regulating role in folliculogenesis; however, knowledge is limited. It is known to stimulate FSH secretion and has inverse associations with serum Inhibin B, estradiol, and P4, yet it is not known to be associated with the gene expression of important granulosa cell hormone receptors [35]. Our study aimed to establish any predictive ability that activin A may have in fecundability based on what is known of its role in the ovary. This is the first study to our knowledge to directly study the correlation of activin A to pregnancy outcomes in rhesus monkeys, a valuable NHP model of women's reproductive health. In this pilot study, it was found that that activin A had no impact on early pregnancy success; however, additional investigation is needed to further establish this in the literature.
In the present study a negative correlation between age and body weight with AMH exists, which is consistent with the literature in humans [1,36-38] and other model species [39,40]. As a female ages, AMH decreases until menopause is reached and AMH production is absent. The impact of obesity on female reproduction is widely acknowledged, albeit a complex process that has not been fully elucidated [41]. Yet, it is known that obesity has a profound impact on hormone secretion and metabolism, which leads to impaired folliculogenesis and follicular atresia [41]. Therefore, it is expected that these two hormones of interest would decrease as weight increased. As with AMH, there was a negative correlation between weight and activin A; however, this was only significant in the animals that became pregnant. This was an unexpected finding, since the data were not significant in the entire cohort nor in females who failed to become pregnant in that cycle (Figure S6). It is worth noting that there are two extreme outliers present in this data set; if they are excluded, the data lose significance. There is some emerging evidence suggesting activin A is elevated in late pregnancy in women just before a diagnosis of preeclampsia [42], but prior to late gestation, activin A levels are unchanged. However, this previous study reported data from samples obtained later in gestation and were likely of placental and not ovarian origin, whereas levels reported in this study were measured in monkeys prior to mating, before placentation. There is a possibility, however, that activin A production may be regulated by metabolic processes, and these outliers were associated with differences in body weight as both were on the lower end of the weight spectrum.
As previously noted, both AMH and activin A are secreted early during folliculogenesis. In our study design, we collected the blood samples at approximately day 7 post-menses. This is a potential limitation to our study, as the collection may have occurred too late in the cycle period, affecting the data we observed. At day 7 in a cycle, both AMH and activin A would begin to decline [7,16]. Samples should be collected around days 1 to 3 post menses to obtain hormone levels when they are at their peak. However, this collection time was a result of the TMB protocol, and this study was limited by the amount of blood allowed to be drawn from the animals. Further limitations could be a result of the sample size used, which may limit the ability to detect significant predictive power for AMH and activin A. Unfortunately, we were constrained by the number of breeding females in the colony; other studies in cattle and women have needed several hundred individuals to reach statistical significance in AMH concentrations between free-breeding or infertile populations not subjected to ART such as ovarian stimulation and embryo transfer.
In this research colony-based study design we did not track live birth rates. This is due to the fact that these animals were assigned to various research studies, some of which involved treatments later in gestation. Because those treatments could impact ongoing live birth rate, we did not analyze AMH or activin A levels later during gestation or correlate with live birth rate. Additional studies in a dedicated cohort of females would be necessary to generate these types of data for further analyses.
Based upon these findings, future work should involve measuring AMH levels on animals undergoing ovarian stimulation specifically, to determine the predictive ability of AMH solely for infertility treatment purposes. Samples should be taken around days 1 to 3 post menses. Another area of future exploration is to supplement animals undergoing ovarian stimulation with AMH to evaluate the impacts of this on folliculogenesis and if oocyte production increases. It would also be of interest to further investigate the relationship between weight and activin A, and the significance that occurred in only the cycles which resulted in a pregnancy. These studies have the potential to further elucidate the impact of obesity on reproductive hormones and folliculogenesis.
5 Conclusion
This study intended to reveal the potential clinical and investigative uses of AMH and activin A, to effectively develop treatments and inform reproductive protocols. While AMH is commonly used clinically, it may not be as reliable as is believed. According to this study, both AMH and activin A are unable to predict the ability to conceive naturally in rhesus monkeys undergoing timed mating protocols. These data are in agreement with a human observational study, where AMH values were not correlated with samples collected early in pregnant cycles [43]. Using AMH as a single snapshot of a woman's ovarian status may produce inaccurate predictions, unless ovarian stimulation and oocyte retrieval are performed. Further investigation is required to assess its ability and use when developing a treatment plan for those undergoing ART treatments; however, caution must be used. Additionally, assessing these reproductive hormones may not be helpful to improve research techniques in reproductive studies.
Back Matter
Acknowledgments
The authors would like to thank the Oregon National Primate Center Behavioral Services Unit for the care and treatment of the animals used in the study. The authors thank Shally Wolf for her technical assistance and help in the lab. Lastly, a special thanks to Timothy Hazzard, PhD, and Katharine Field, PhD, for assisting and editing the manuscript.
Conflicts of Interest
C. Bishop was a paid consultant for Oviva Therapeutics, and collaborates on projects supported by 21st Century Medicine and Expanse Bio, LLC. All other authors declare no conflicts of interest.
Author Contributions
M.R. was involved in data collection, performed data analyses, as well as writing and editing manuscript. J.J. was involved in sample preparation and identification, and manuscript approval. C.B.H. and J.X. were involved in experimental design, sample preparation, data interpretation, and manuscript approval. C.V.B was involved in all aspects of the study including the experimental design, data collection, data analysis, and data interpretation as well as writing and editing of the manuscript.
Ethics Approval and Consent to Participate
All animal procedures were approved by the Oregon Health & Science University (OHSU), Oregon National Primate Research Center (ONPRC) Institutional Animal Care and Use Committee (IACUC) and comply with the Animal Welfare Act and the Guide for the Care and Use of Laboratory Animals (The Guide; https://doi.org/10.17226/12910).
Funding
This research was supported by the National Institutes of Health (NIH) Office of the Director P51OD011092 (CBH). The content is solely the responsibility of the authors and does not necessarily represent the official views of the NIH. Additional support for CVB provided by the USDA National Institute of Food and Agriculture and Multistate/Regional Research and/or Extension Appropriations Multistate Research Project #NE2227.
Availability of Data and Materials
All summarized data supporting this study are included within the article and supporting materials. Full datasets are available upon reasonable request from the corresponding author.
Supplementary Materials
The following supporting information can be downloaded at: https://ojs.exploverpub.com/index.php/jecacm/article/view/215/sup. Figure S1. A representation of the pregnancy outcomes in the cohort of cycles that were used in the study. Figure S2. Representation of the day of the cycle in which the first blood sample was taken that was utilized in hormone measuring of AMH and activin A. Figure S3. Box plot analyses of impact of age (years) on cycle outcomes (pregnant vs not pregnant, p > 0.12). Figure S4. Box plot analyses of impact of weight (kg) on cycle outcomes (pregnant vs not pregnant, p > 0.36). Figure S5. Correlation analyses between serum AMH concentration prior to breeding (pg/mL) versus either age (years) or weight (kg) as separated by cycles resulting in a Pregnancy (Panel A, top graphs) or not (Non-Pregnant Cycle; Panel B, bottom graphs). Figure S6. Correlation analyses between serum Activin A (pg/mL) concentration prior to breeding (pg/mL) versus either age (years) or weight (kg) as separated by cycles resulting in a Pregnancy or not (Non-Pregnant Cycles), as compared with combining analyses from all cycles.
References
- Visser JA, Schipper I, Laven JS, et al. Anti-Müllerian hormone: an ovarian reserve marker in primary ovarian insufficiency. Nature Reviews. Endocrinology 2012; 8(6): 331-341.
- Broekmans FJ, Soules MR, Fauser BC. Ovarian aging: mechanisms and clinical consequences. Endocrine Reviews 2009; 30(5): 465-493.
- Park SU, Walsh L, Berkowitz KM. Mechanisms of ovarian aging. Reproduction (Cambridge, England) 2021; 162(2): R19-R33.
- Tal R, Seifer DB. Ovarian reserve testing: a user's guide. American Journal of Obstetrics and Gynecology 2017; 217(2): 129-140.
- Gizzo S, Andrisani A, Esposito F, et al. Ovarian Reserve Test: An Impartial Means to Resolve the Mismatch Between Chronological and Biological Age in the Assessment of Female Reproductive Chances. Reproductive Sciences (Thousand Oaks, Calif.) 2014; 21(5): 632-639.
- Shahrokhi SZ, Kazerouni F, Ghaffari F. Anti-Müllerian Hormone: genetic and environmental effects. Clinica Chimica Acta; International Journal of Clinical Chemistry 2018; 476: 123-129.
- Salmon NA, Handyside AH, Joyce IM. Oocyte regulation of anti-Müllerian hormone expression in granulosa cells during ovarian follicle development in mice. Developmental Biology 2004; 266(1): 201-208.
- Durlinger AL, Gruijters MJ, Kramer P, et al. Anti-Mullerian hormone inhibits initiation of primordial follicle growth in the mouse ovary. Endocrinology 2002; 143(3): 1076-1084.
- Knauff EA, Eijkemans MJ, Lambalk CB, et al. Anti-Mullerian hormone, inhibin B, and antral follicle count in young women with ovarian failure. The Journal of Clinical Endocrinology and Metabolism 2009; 94(3): 786-792.
- van Beek RD, van den Heuvel-Eibrink MM, Laven JS, et al. Anti-Mullerian hormone is a sensitive serum marker for gonadal function in women treated for Hodgkin's lymphoma during childhood. The Journal of Clinical Endocrinology and Metabolism 2007; 92(10): 3869-3874.
- Nelson SM, Fleming R, Gaudoin M, et al. Antimüllerian hormone levels and antral follicle count as prognostic indicators in a personalized prediction model of live birth. Fertility and sterility 2015; 104(2): 325-332.
- Ribeiro ES, Bisinotto RS, Lima FS, et al. Plasma anti-Müllerian hormone in adult dairy cows and associations with fertility. Journal of Dairy Science 2014; 97(11): 6888-6900.
- Steiner AZ, Pritchard D, Stanczyk FZ, et al. Association between biomarkers of ovarian reserve and infertility among older women of reproductive age. JAMA 2017; 318(14): 1367-1376.
- Adu-Gyamfi EA, Djankpa FT, Nelson W, et al. Activin and inhibin signaling: from regulation of physiology to involvement in the pathology of the female reproductive system. Cytokine 2020; 133: 155105.
- Matzuk MM. Revelations of ovarian follicle biology from gene knockout mice. Molecular and Cellular Endocrinology 2000; 163(1-2): 61–66.
- Cossigny DA, Findlay JK, Drummond AE. The effects of FSH and activin A on follicle development in vitro. Reproduction (Cambridge, England) 2012; 143(2): 221.
- Saravanan C, Flandre T, Hodo CL, et al. Research relevant conditions and pathology in nonhuman primates. ILAR Journal 2020; 61(2-3): 139-166.
- Stouffer RL, Woodruff TK. Nonhuman primates: a vital model for basic and applied research on female reproduction, prenatal development, and women's health. ILAR Journal 2017; 58(2): 281-294.
- Tardif S, Carville A, Elmore D, et al. Reproduction and breeding of nonhuman primates. Nonhuman Primates in Biomedical Research 2012: 197.
- Walker ML, Herndon JG. Menopause in nonhuman primates? Biology of Reproduction 2008; 79(3): 398-406.
- Wolf DP. Artificial insemination and the assisted reproductive technologies in non-human primates. Theriogenology 2009; 71(1): 123-129.
- Bishop CV, Stouffer RL, Takahashi DL, et al. Chronic hyperandrogenemia and western-style diet beginning at puberty reduces fertility and increases metabolic dysfunction during pregnancy in young adult, female macaques. Human reproduction (Oxford, England) 2018; 33(4): 694-705.
- McGee WK, Bishop CV, Bahar A, et al. Elevated androgens during puberty in female rhesus monkeys lead to increased neuronal drive to the reproductive axis: a possible component of polycystic ovary syndrome. Human reproduction (Oxford, England) 2012; 27(2): 531-540.
- Xu J, Xu F, Lawson MS, et al. Anti-Müllerian hormone is a survival factor and promotes the growth of rhesus macaque preantral follicles during matrix-free culture. Biology of Reproduction 2018; 98(2): 197-207.
- Nichols SM, Bavister BD, Brenner CA, et al. Ovarian senescence in the rhesus monkey (Macaca mulatta). Human reproduction (Oxford, England) 2005; 20(1): 79-83.
- Kubisch HM, Falkenstein KP, Deroche CB, et al. Reproductive efficiency of captive Chinese- and Indian-origin rhesus macaque (Macaca mulatta) females. American journal of Pimatology 2012; 74(2): 174-184.
- Simopoulou M, Sfakianoudis K, Antoniou N, et al. Making IVF more effective through the evolution of prediction models: is prognosis the missing piece of the puzzle? Systems Biology in Reproductive Medicine 2018; 64(5): 305-323.
- Broer SL, Broekmans FJ, Laven JS, et al. Anti-Müllerian hormone: ovarian reserve testing and its potential clinical implications. Human reproduction update 2014; 20(5): 688-701.
- Hvidman HW, Bentzen JG, Thuesen LL, et al. Infertile women below the age of 40 have similar anti-Müllerian hormone levels and antral follicle count compared with women of the same age with no history of infertility. Human Reproduction (Oxford, England) 2016; 31(5): 1034-1045.
- Zarek SM, Mitchell EM, Sjaarda LA, et al. Is anti-Müllerian hormone associated with fecundability? Findings from the EAGeR trial. The Journal of Clinical Endocrinology and Netabolism 2015; 100(11): 4215-4221.
- Lin C, Jing M, Zhu W, et al. The value of anti-Müllerian hormone in the prediction of spontaneous pregnancy: a systematic review and meta-analysis. Frontiers in Endocrinology 2021; 12: 695157.
- Pacchiarotti A, Iaconianni P, Caporali S, et al. Severe endometriosis: low value of AMH did not affect oocyte quality and pregnancy outcome in IVF patients. European Review for Medical and Pharmacological Sciences 2020; 24(22): 11488-11495.
- Takahashi C, Fujito A, Kazuka M, et al. Anti-Müllerian hormone substance from follicular fluid is positively associated with success in oocyte fertilization during in vitro fertilization. Fertility and sterility 2008; 89(3): 586-591.
- Bosch E, Nyboe Andersen A, Barri P, et al. Follicular and endocrine dose responses according to anti-Müllerian hormone levels in IVF patients treated with a novel human recombinant FSH (FE 999049). Clinical Endocrinology 2015; 83(6): 902-912.
- Jeppesen JV, Nielsen ME, Kristensen SG, et al. Concentration of activin A and follistatin in follicular fluid from human small antral follicles associated to gene expression of the corresponding granulosa cells. Molecular and cellular endocrinology 2012; 356(1-2): 48-54.
- de Vet A, Laven JSE, de Jong FH, et al. Antimüllerian hormone serum levels: a putative marker for ovarian aging. Fertility and Sterility 2002; 77(2): 357-362.
- van Rooij IA, Broekmans FJ, Scheffer GJ, et al. Serum antimüllerian hormone levels best reflect the reproductive decline with age in normal women with proven fertility: a longitudinal study. Fertility and Sterility 2005; 83(4): 979-987.
- Ramezani Tehrani F, Bidhendi Yarandi R, Solaymani-Dodaran M, et al. Improving Prediction of Age at Menopause Using Multiple Anti-Müllerian Hormone Measurements: the Tehran Lipid-Glucose Study. The Journal of Clinical Endocrinology and Metabolism 2020; 105(5): 1589-1598.
- Hou H-Y, Wang X, Yu Q, et al. Evidence that growth hormone can improve mitochondrial function in oocytes from aged mice. Reproduction (Cambridge, England) 2019; 157(4): 345-358.
- Downs JL, Urbanski HF. Neuroendocrine changes in the aging reproductive axis of female rhesus macaques (Macaca mulatta). Biology of Reproduction 2006; 75(4): 539-546.
- Talmor A, Dunphy B. Female obesity and infertility. Best Practice & Research. Clinical Obstetrics & Gynaecology 2015; 29(4): 498-506.
- Wong GP, Andres F, Walker SP, et al. Circulating Activin A is elevated at 36 weeks' gestation preceding a diagnosis of preeclampsia. Pregnancy Hypertension 2022; 27: 23-26.
- Streuli I, de Mouzon J, Paccolat C, et al. AMH concentration is not related to effective time to pregnancy in women who conceive naturally. Reproductive Biomedicine Online 2014; 28(2): 216-224.