Main Text
1 Introduction
Stroke is one of the top three diseases in terms of mortality globally and has nearly 6 million deaths annually, a number that is expected to reach 12 million by 2030 [1]. Post-stroke depression (PSD) is defined as depression state after the onset of stroke [1], which is a severe complication of stroke and has gradually become one of the commonest neuropsychological complications of stroke, resulting in declined life quality and rehabilitation ability. PSD can occur in the acute, recovery, and sequelae stages of stroke, and during difference follow-up periods, the incidence of PSD ranges from 20% to 43% [2]. The primary clinical symptoms of PSD include low mood, loss of interest, emptiness, anxiety, irritability, pessimism, sleep disorders, general fatigue, etc. [3,4]. There are multiple causes for the occurrence of PSD, such as female, age (<70 years), family history, nervousness, severity of stroke and disability, anxiety, aphasia, cognitive ability, and chronic stress (including financial and health-related stress) [5,7]. Also, PSD is an important factor for the recurrence of stroke, which not only seriously affects the quality of life of patients, but also imposes a serious burden on stroke families and society.
Currently, the underlying physiological and pathological mechanism of PSD remains unclear [8], but the possible mechanisms consist of changes in the upstream monoamine pathway, excessive pro-inflammatory cytokines, dysfunction of the hypothalamic-pituitary-adrenal (HPA) axis, and alterations in neuroplasticity according to heaps of research [1]. The treatment of PSD mainly comprises selective serotonin reuptake inhibitors (SSRIs), serotonin and norepinephrine reuptake inhibitors (SNRIs), and other antidepressants [1]. However, these antidepressants have multiple side effects, such as dizziness, drowsiness, and anticholinergic side effects [9]. Moreover, there exists some controversy over the effectiveness of antidepressants in improving the quality of life, function, and cognitive outcomes of patients with neurological disorders [10]. To minimize side effects and maximize therapeutic efficacy, alternative treatment methods are sorely needed [11].
From the perspective of traditional Chinese medicine (TCM), PSD belongs to concurrent meridian pattern of "wind stroke" and "qi stagnation", which, based on wind stroke, is a depressive manifestation resulting from blood stasis and qi stagnation, as well as obstructed qi in liver due to the stagnation of wind, phlegm, fire and blood stasis [12]. Hence, despite the disease location in the brain, PSD has the closest relationship with the liver [13]. "Radix Bupleuri-Paeoniae Radix Alba" is the most fundamental medicine pair for "soothing the liver, resolving depression, and regulating emotions", which forms the basis of formulas such as Xiao Yao San, Si Ni San, and Xiao Chai Hu Tang in the Bupleurum category. A previous study explored the medication rules of TCM for PSD on the Chinese medicine inheritance auxiliary platform and found that "Radix Bupleuri-Paeoniae Radix Alba" is one of the top five most frequently used drug combinations targeting PSD [14]. Radix Bupleuri is a commonly used drug for soothing the liver, and the Transforming the Significance of Medicinal Substances recorded that "Radix Bupleuri is light and clear in nature governing ascending and dispersing, and is slightly bitter in taste mainly for soothing the liver" [15]. Paeoniae Radix Alba is bitter and sour in taste, and slightly cold in nature, which can nourish blood, consolidate Yin, soften the liver, and relieve pain. The combination of these two drugs has the function of dispersing and consolidating, and nourishing both qi and blood, exerting synergetic effects [16]. Modern pharmacological studies have shown that Radix Bupleuri has pharmacological effects such as antipyretic, analgesic, anti-inflammatory, and antidepressant properties [17], while Paeoniae Radix Alba possesses analgesic, anti-inflammatory, antidepressant, anti-cardiovascular/cerebrovascular diseases, and anti-neurodegenerative diseases effects [18]. Reportedly, the Radix Bupleuri-Paeoniae Radix Alba combination is significantly effective in treating PSD [19], but the relevant specific material basis and mechanism are not yet clarified. This study utilizes network pharmacology analysis to screen the core active ingredients, key targets, and main signaling pathways of "Radix Bupleuri-Paeoniae Radix Alba" in treating PSD, and leverages molecular docking methods to verify the binding of active ingredients and key targets, to explore the main material basis and mechanism of "Radix Bupleuri-Paeoniae Radix Alba" in treating PSD, providing a specific basis for future in-depth research.
2 Materials and methods
2.1 Screening of active ingredients in Radix Bupleuri-Paeoniae Radix Alba and targets for PSD
Screening of active ingredients and targets of Radix Bupleuri-Paeoniae Radix Alba: All effective active ingredients of Radix Bupleuri-Paeoniae Radix Alba were retrieved on Traditional Chinese Medicine Systems Pharmacology (TCMSP) (https://tcmspw.com/tcmsp.php), with the settings of oral bioavailability (OB) value ≥ 30% and drug-likeness (DL) ≥ 0.18. The target prediction of the included compound ingredients was conducted using TCMSP database, and all targets were corrected and converted to symbol name using the Uniprot database (https://www.uniprot.org/) with non-human targets removed.
Screening of targets of PSD: With "Post stroke depression" as key words, relevant targets of PSD were searched in GeneCards (https://www.genecards.org/), NCBI (https://www.ncbi.nlm.nih.gov/) and DisGeNET (https://www.disgenet.org/) databases. The results from the three databases were integrated and the duplicated gene targets were deleted, yielding PSD-related targets.
2.2 Construction of intersection of drug and disease-related targets
To further anatomize the relation between "Radix Bupleuri-Paeoniae Radix Alba" and PSD, the selected drug targets and disease targets were input in Venn 2.1 for drawing a Venn diagram of the relationship between "Radix Bupleuri-Paeoniae Radix Alba" and PSD-related targets. The common targets were identified and acted as predictive targets for the effect of "Radix Bupleuri-Paeoniae Radix Alba" on PSD.
2.3 Construction of "TCM-active ingredients-disease-intersection targets" network and screening of core active ingredients
With the obtained intersection targets of drug and disease in Section 2.2 and through collating TCM, active ingredients and disease information, Cytoscape 3.8.0 was exploited to establish "TCM-active ingredients-disease-intersection targets" network [20]. Topology analysis was performed using Network Analyzer tool and the core ingredients of "Radix Bupleuri-Paeoniae Radix Alba" for treating PSD were selected based on topology parameters.
2.4 Construction of protein-protein interaction (PPI) network diagram and screening of core proteins
The common targets of drug and diseases were input into the String database (https://string-db.org/cgi/input.pl) for constructing a PPI network [21]. With "Homo sapiens" as biological species. The hide disconnected nodes were removed from the network and the minimum required interaction score was set as high confidence of 0.900 to get the data of PPI. Then, the data of PPI was imported into Cytoscape to draw the PPI network, and the top 10 core proteins were obtained by R 4.1. Core targets were filtered through three key topological parameters, including degree centrality (DC), closeness centrality (CC) and betweenness centrality (BC). The values of these three parameters indicate the importance and influence of related nodes in the network. The cutoff value was set to the median. PPI network were filtered by molecular complex detection algorithm (MCODE) plugin in Cytoscape. Herein, degree was set to 2, k-core was set to 2, max depth was set to 0.2, and maximum depth was set to 100.
2.5 GO function and KEGG pathway enrichment analysis
The common targets of drug and diseases underwent KEGG pathway enrichment analysis and GO function enrichment analysis from three levels of biological process (BP), molecular function (MF) and cell component (CC).
Utilizing the String database, the items with a corrected p value of less than 0.05 were filtered. With R 4.0.3 software and the packages clusterProfiler, enrichplot, and ggplot2 installed, the bar and bubble charts were plotted. To more vividly illustrate the multi-ingredient and multi-target characteristics of active ingredients in TCM during the treatment process, the ingredient-disease-pathway-target network files were imported into Cytoscape 3.8.0 for the generation of pathway network diagrams.
2.6 Molecular docking of key targets and core active ingredients
3D structure of core proteins in pathway was retrieved in RCSB Protein Data Bank database (https://www.rcsb.org/). Through the Pubchem database (https://pubchem.ncbi.nlm.nih.gov/), the structural file of the main active ingredients in SDF format was downloaded and imported into Open Babel 2.4.1 software to convert 2D small molecule compounds into 3D structures in mol2 format. Using AutoDock Tools 1.5.6 software, the proteins and small molecule compounds underwent dehydration and hydrogenation, the charge was calculated, and non-polar hydrogen was combined. The results were visualized in PyMOL Molecular Graphics System software.
3 Results
3.1 Screening of active ingredients and targets in Radix Bupleuri-Paeoniae Radix Alba
Due to multi-ingredient and multi-target characteristics of TCM, it is necessary to filter active compounds. After summarizing and deleting duplicates through retrieval in TCMSP database and absorption, distribution, metabolism and excretion (ADME) parameter screening, we obtained 17 compound ingredients and 179 targets of Radix Bupleuri, as well as 13 compound ingredients and 86 targets of Paeoniae Radix Alba. Among them, kaempferol is an active ingredient both existing in Radix Bupleuri and Paeoniae Radix Alba and there were a total of 29 active ingredients of "Radix Bupleuri-Paeoniae Radix Alba" (Table 1).
Table 1 Active ingredients of Radix Bupleuri and Paeoniae Radix Alba.
Traditional Chinese medicine | No. | Name | OB/% | DL |
---|---|---|---|---|
Radix Bupleuri | MOL001645 | Linoleyl acetate | 42.10 | 0.20 |
Radix Bupleuri | MOL002776 | Baicalin | 40.12 | 0.75 |
Radix Bupleuri | MOL000449 | Stigmasterol | 43.83 | 0.76 |
Radix Bupleuri | MOL000354 | isorhamnetin | 49.60 | 0.31 |
Radix Bupleuri | MOL004598 | 3,5,6,7-tetramethoxy-2-(3,4,5-trimethoxyphenyl)chromone | 31.97 | 0.59 |
Radix Bupleuri | MOL004609 | Areapillin | 48.96 | 0.41 |
Radix Bupleuri | MOL013187 | Cubebin | 57.13 | 0.64 |
Radix Bupleuri | MOL004624 | Longikaurin A | 47.72 | 0.53 |
Radix Bupleuri | MOL004628 | Octalupine | 47.82 | 0.28 |
Radix Bupleuri | MOL004644 | Sainfuran | 79.91 | 0.23 |
Radix Bupleuri | MOL004648 | Troxerutin | 31.60 | 0.28 |
Radix Bupleuri | MOL004653 | (+)-Anomalin | 46.06 | 0.66 |
Radix Bupleuri | MOL004702 | saikosaponin c_qt | 30.50 | 0.63 |
Radix Bupleuri | MOL004718 | α-spinasterol | 42.98 | 0.76 |
Radix Bupleuri | MOL000490 | petunidin | 30.05 | 0.31 |
Radix Bupleuri | MOL000098 | quercetin | 46.43 | 0.28 |
Radix Bupleuri and Paeoniae Radix Alba | MOL000422 | kaempferol | 41.88 | 0.24 |
Paeoniae Radix Alba | MOL000492 | (+)-catechin | 54.83 | 0.24 |
Paeoniae Radix Alba | MOL001928 | albiflorin_qt | 66.64 | 0.33 |
Paeoniae Radix Alba | MOL001918 | paeoniflorgenone | 87.59 | 0.37 |
Paeoniae Radix Alba | MOL001910 | 11alpha,12alpha-epoxy-3beta-23-dihydroxy-30-norolean-20-en-28,12beta-olide | 64.77 | 0.38 |
Paeoniae Radix Alba | MOL001925 | paeoniflorin_qt | 68.18 | 0.4 |
Paeoniae Radix Alba | MOL001919 | (3S,5R,8R,9R,10S,14S)-3,17-dihydroxy-4,4,8,10,14-pentamethyl-2,3,5,6,7,9-hexahydro-1H-cyclopenta[a]phenanthrene-15,16-dione | 43.56 | 0.53 |
Paeoniae Radix Alba | MOL001930 | benzoyl paeoniflorin | 31.27 | 0.75 |
Paeoniae Radix Alba | MOL000359 | sitosterol | 36.91 | 0.75 |
Paeoniae Radix Alba | MOL000358 | beta-sitosterol | 36.91 | 0.75 |
Paeoniae Radix Alba | MOL000211 | Mairin | 55.38 | 0.78 |
Paeoniae Radix Alba | MOL001924 | paeoniflorin | 53.87 | 0.79 |
Paeoniae Radix Alba | MOL001921 | Lactiflorin | 49.12 | 0.80 |
3.2 Information about PSD-related targets and obtainment of "TCM-disease" intersection targets
The retrieval on GeneCards, NCBI and DisGeNET databases revealed 2535, 151 and 39 PSD-related targets, respectively. After we integrated, de-duplicated and standardized the genes from these three databases, a total of 2544 PSD-related targets were obtained. The "Radix Bupleuri-Paeoniae Radix Alba" targets and disease targets were imported into Venn 2.1, resulting in 145 common targets, which were potential targets in treatment of PSD using "Radix Bupleuri-Paeoniae Radix Alba" medicine pair (Figure 1).

Figure 1 The Venn diagram of "Radix Bupleuri-Paeoniae Radix Alba" medicine pair and PSD targets.
3.3 Construction of "TCM-active ingredients-disease-intersection targets" network and screening of core active ingredients
To unveil the intersection between targets of "Radix Bupleuri-Paeoniae Radix Alba" active ingredients and disease targets, information regarding TCM (Radix Bupleuri-Paeoniae Radix Alba), active ingredients, intersection targets and disease was imported into Cytoscape 3.8.0, constructing "TCM-active ingredients-disease-intersection targets" network (Figure 2). In the figure, the blue purple represents the active ingredients, the green represents the target of the drug acting on the disease, the yellow represents the "Radix Bupleuri-Paeoniae Radix Alba" medicine pair, and the red rectangle represents the disease. The ingredients were sorted by degree, with higher degree values indicating greater importance of the ingredients (Table 2). From Table 2, it can be noticed that the top three targets with high correlation were quercetin, kaempferol and β-sitosterol, among which kaempferol was a common component of Radix Bupleuri and Paeoniae Radix Alba. In Radix Bupleuri, quercetin had the strongest correlation with the target, while in Paeoniae Radix Alba, β-sitosterol had the strongest association with target. Overall, quercetin and kaempferol had the most interactions with their targets and may have relatively greater value for future research.
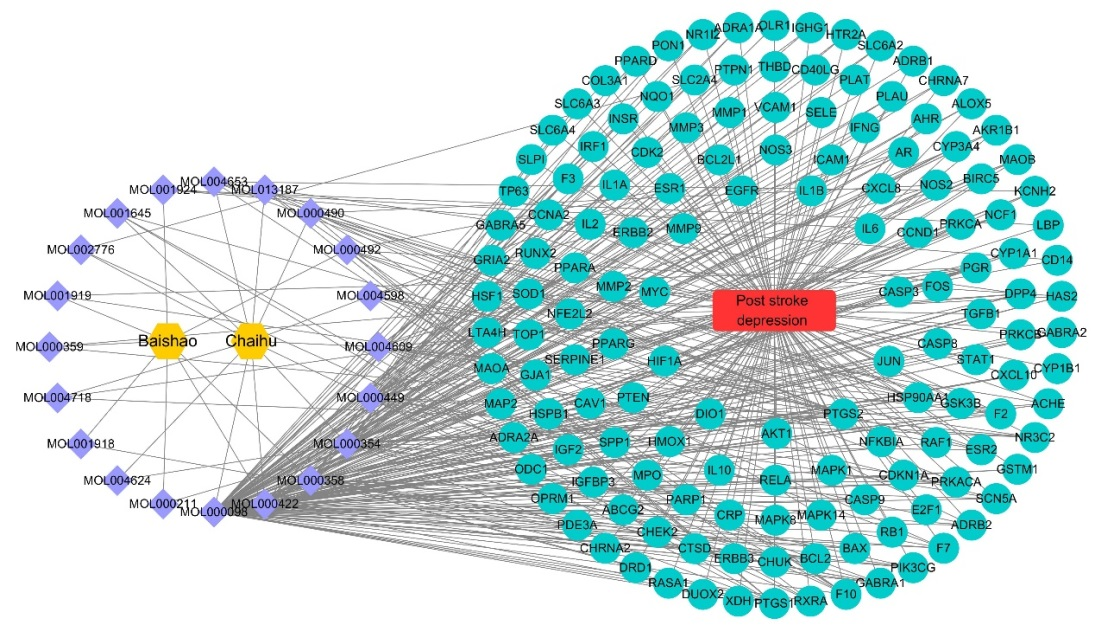
Figure 2 The "TCM-active ingredients-disease-intersection targets" network of "Radix Bupleuri-Paeoniae Radix Alba" in the treatment of PSD.
Table 2 The core active ingredients of "Radix Bupleuri-Paeoniae Radix Alba" in the treatment of PSD.
MOL ID | Name | Degree |
---|---|---|
MOL000098 | quercetin | 111 |
MOL000422 | kaempferol | 44 |
MOL000358 | beta-sitosterol | 31 |
MOL000354 | isorhamnetin | 28 |
MOL000449 | Stigmasterol | 23 |
MOL004609 | Areapillin | 13 |
MOL004598 | 3,5,6,7-tetramethoxy-2-(3,4,5-trimethoxyphenyl)chromone | 9 |
MOL000490 | petunidin | 8 |
MOL000492 | (+)-catechin | 8 |
MOL004653 | (+)-Anomalin | 6 |
MOL013187 | Cubebin | 6 |
MOL001645 | Linoleyl acetate | 4 |
MOL001924 | paeoniflorin | 4 |
MOL000359 | sitosterol | 3 |
MOL001919 | (3S,5R,8R,9R,10S,14S)-3,17-dihydroxy-4,4,8,10,14-pentamethyl-2,3,5,6,7,9-hexahydro-1H-cyclopenta[a]phenanthrene-15,16-dione | 3 |
MOL002776 | Baicalin | 3 |
MOL004718 | α-spinasterol | 3 |
MOL000211 | Mairin | 2 |
MOL001918 | paeoniflorgenone | 2 |
MOL004624 | Longikaurin A | 2 |
3.4 Construction of PPI network and screening of core proteins
The potential targets of "Radix Bupleuri-Paeoniae Radix Alba" in the treatment of PSD were imported into String database for PPI network construction, with "Homo sapiens" as biological species (Figure 3). The PPI network had 145 nodes, 2378 edges, and mean degree of 33. The PPI network was imported into Cytoscape 3.8.0 to perform topology analysis using the Network Analyzer tool, and the top 20 target genes with the most linked nodes were sorted out to create a bar diagram of the core genes in the PPI network (Figure 4). The core protein target genes included AKT1, IL-6, IL-1β, etc., which provided certain reference value for studying the mechanism of "Radix Bupleuri-Paeoniae Radix Alba" in treating PSD.

Figure 3 PPI network diagram.
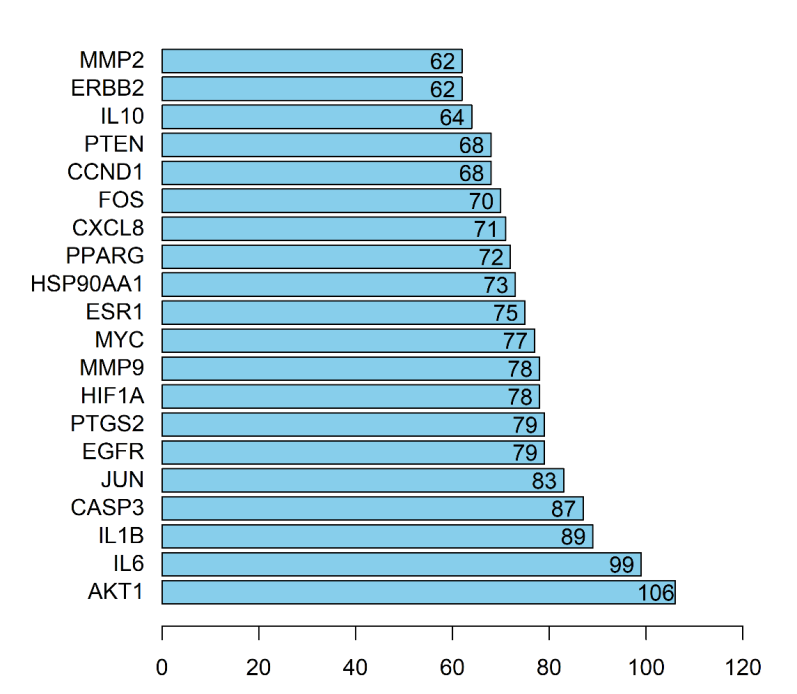
Figure 4 PPI network of proteins of core genes.
3.5 GO function and KEGG pathway enrichment analysis
To clarify the mechanism of active ingredients of "Radix Bupleuri-Paeoniae Radix Alba" in the treatment of PSD, we obtained GO function bar diagram (Figure 5) and KEGG pathway enrichment bubble diagram (Figure 6) based on R language. The results revealed that the main enrichment items of potential targets comprised "response to lipopolysaccharide", "response to molecule of bacterial origin", "cellular response to chemical stress", "response to drug" and "response to oxidative stress" in biological processes; "membrane raft", "membrane microdomain", and "membrane regions" in the cellular component group; and "DNA-binding-transcription factor binding", "RNA polymerase Ⅱ specific DNA-binding-transcription factor binding", "ubiquitin-like protein ligase binding", etc., in molecular functions.
The results indicated that KEGG pathways with high enrichment included "lipids and atherosclerosis", "chemical carcinogenesis-receptor activation", "Hepatitis B", "AGE-RAGE signaling pathway in diabetes complications", "Kaposi sarcoma associated herpesvirus infection". Besides, "Radix Bupleuri-Paeoniae Radix Alba" regulated PSD via multiple biological processes and signaling pathways. We noticed that "lipids and atherosclerosis" ranked first in KEGG pathway enrichment, and reportedly, atherosclerosis is a risk factor for PSD [22].
The "active ingredients-disease-pathway-targets" network of "Radix Bupleuri-Paeoniae Radix Alba" in the treatment of PSD was imported into Cytoscape3.8.0 to establish "TCM-active ingredients-core targets-biological function-signaling pathway" network (Figure 7). As delineated in Figure 7, the blue, pink, green, yellow and purple signify compounds, targets of TCM acting on disease, the most prominent top 20 pathways, diseases, and TCM. The network contributed to visualization of main material basis and mechanism of "Radix Bupleuri-Paeoniae Radix Alba" in the treatment of PSD.
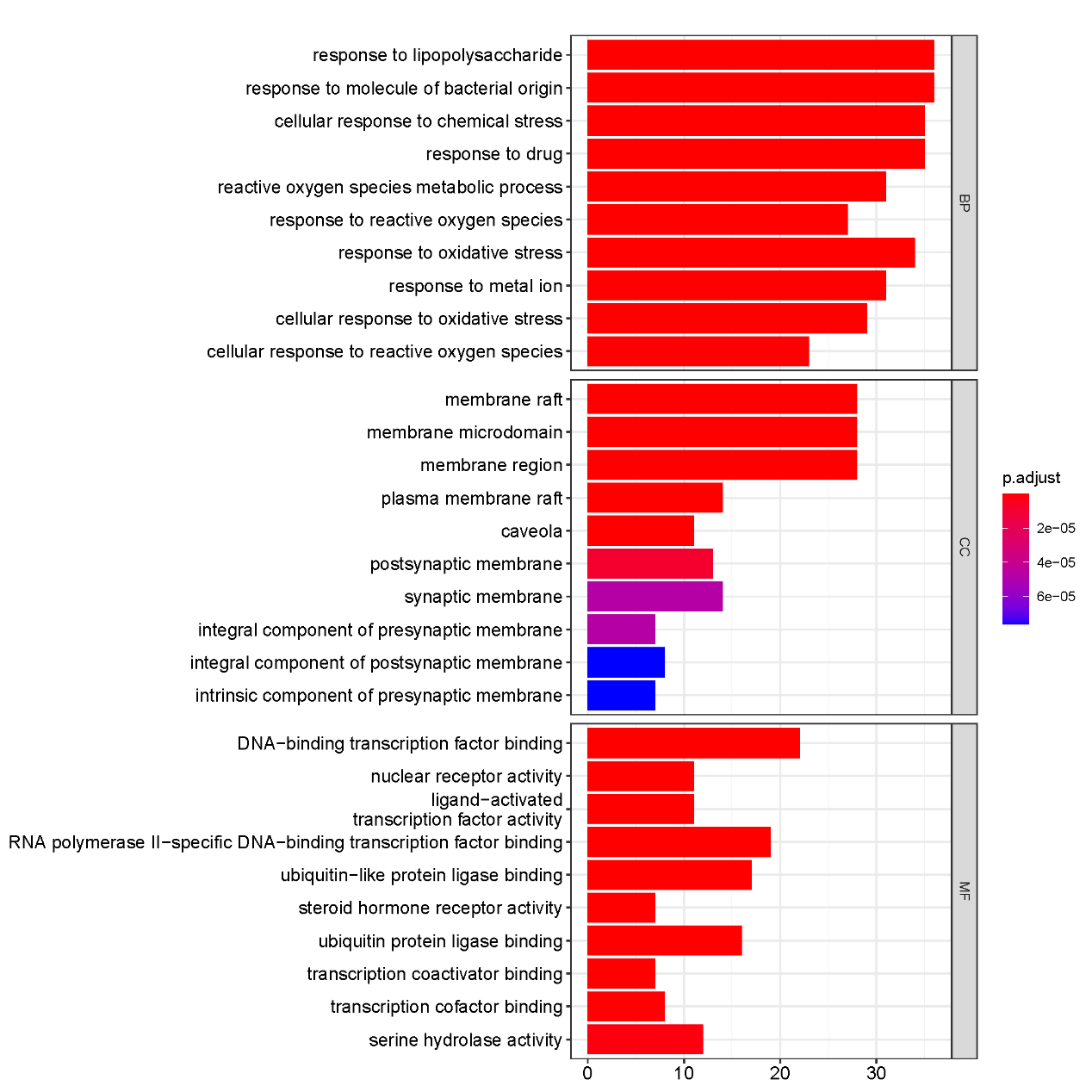
Figure 5 The bar diagram of GO function analysis.
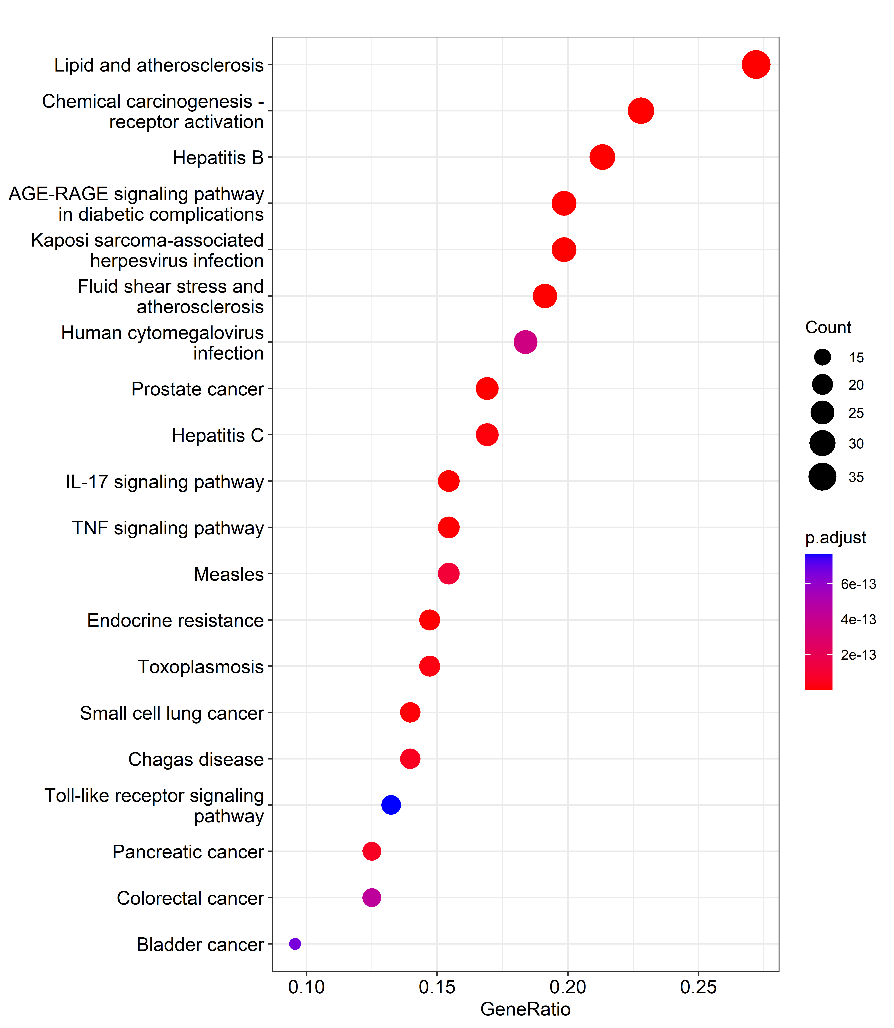
Figure 6 The bubble diagram of KEGG pathway.

Figure 7 The network of "TCM-active ingredients-core targets-biological function-signaling pathway" of "Radix Bupleuri-Paeoniae Radix Alba" in the treatment of PSD.
3.6 Molecular docking
Using Sybyl-X 2.0 software, the top 3 core targets and top 2 core ingredients with the highest degree values were selected for molecular docking (Table 3), with the lower binding energy score implying the more stable binding. We confirmed that the binding energy score of the selected core ingredients and the target docking was between -5.0 and -7.0, indicating that the small molecules of the compound had good binding ability to the target. Among them, kaempferol had a good affinity for AKT1, while quercetin had a good affinity for IL-1β, AKT1, and IL-6 proteins. These findings hinted that quercetin and kaempferol played critical roles in the efficacy of "Radix Bupleuri-Paeoniae Radix Alba", which provided pivotal reference for the mechanism of the medicine pair in PSD treatment.
The schematic diagram of docking of core ingredients with important target protein molecules is shown in Figure 8.
Table 3 Information of molecular docking results.
Protein and PDB ID | Name of compound | Binding energy score (kcal/mol) |
---|---|---|
AKT1(2UVM) | kaempferol | -6.2 |
AKT1(2UVM) | quercetin | -6.5 |
IL-1β(6Y8I) | quercetin | -6.8 |
IL-6(1ALU) | quercetin | -5.7 |
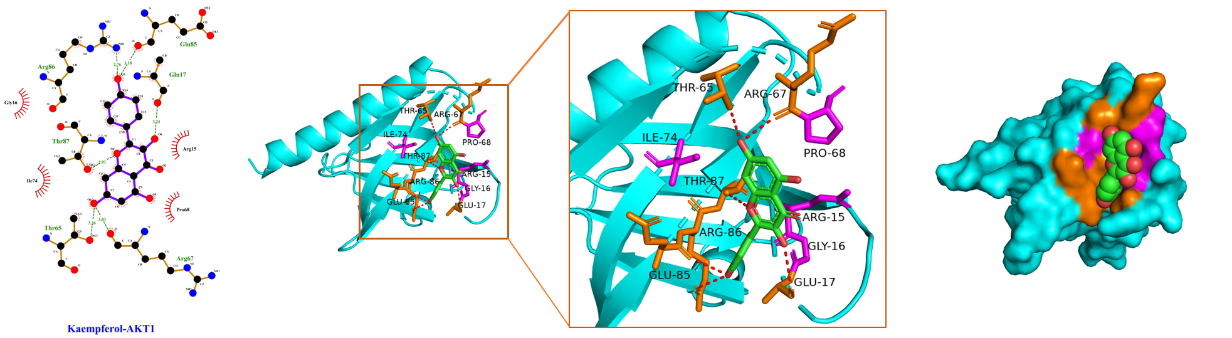
(A) Kaempferol and AKT1
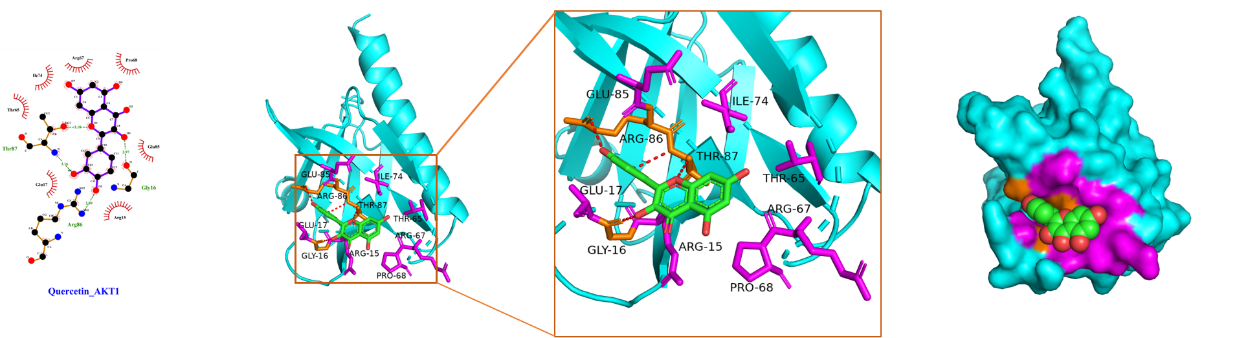
(B) Quercetin and AKT1
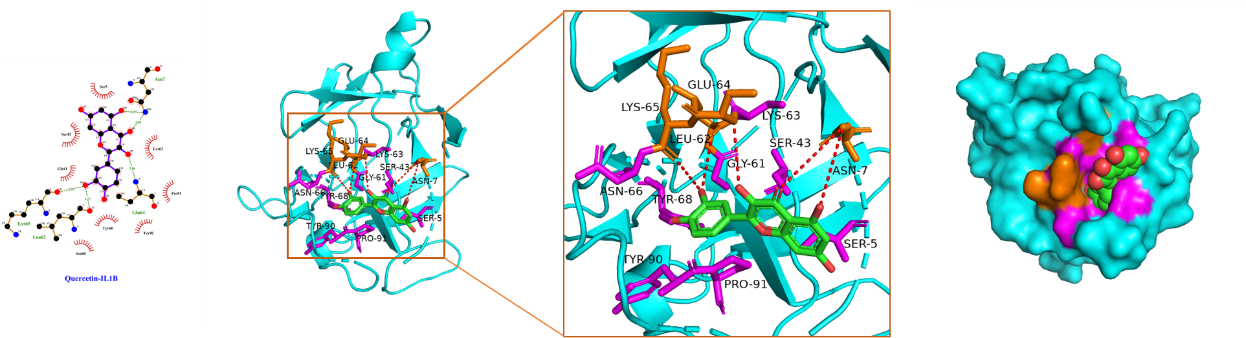
(C) Quercetin and IL-1β
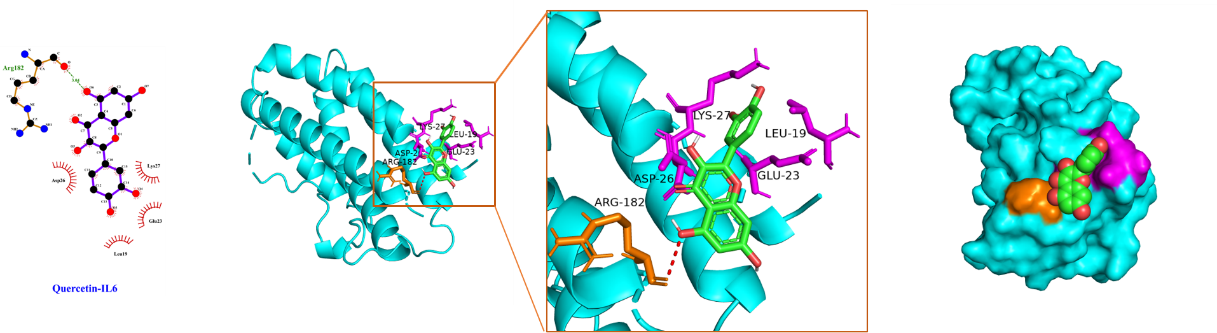
(D) Quercetin and IL-6
Figure 8 Schematic diagram of docking of core ingredients with important target protein molecules.
4 Discussion
PSD is the commonest and severest psychoneurologic complication after stroke, and has the morbidity and disability rate increasing year by year in recent years, impacting nearly one third of stroke patients [23]. The current treatment lacks effective specific methods and mainly depends on antidepressant drugs; however, long-term use of these drugs can lead to a series of problems such as adverse reactions and drug resistance. TCM has the advantages of multi-target, multi-system, multi-channel and small toxic and side effects in treatment of PSD [24].
The relevant research pointed out that Radix Bupleuri-Paeoniae Radix Alba is one of the top five drug pair used most frequently in the treatment of PSD [14]. However, the specific mechanism of active ingredients in Radix Bupleuri-Paeoniae Radix Alba remains undefined currently. This study performed analyses through network model construction, and screened out 29 active ingredients from Radix Bupleuri-Paeoniae Radix Alba and 145 potential targets for PSD treatment. By constructing a drug-active ingredients-potential target network and PPI network, the main ingredients of Radix Bupleuri-Paeoniae Radix Alba for treating PSD were predicted to be quercetin, kaempferol, and β-sitosterol. Among them, quercetin and kaempferol had the most corresponding targets, and the core targets of Radix Bupleuri-Paeoniae Radix Alba for treating PSD included AKT1, IL-6, IL-1β, etc. Previous study have found that Xiaoyao-jieyu-san also improves depression after stroke, and a large number of the active components include quercetin, kaempferol, etc. [25]. Quercetin possesses wide pharmacological effects, such as anti-oxidant, free radical scavenging, anti-inflammation, anti-tumor and neuroprotection [26], and has been reported to have good anti-depression effect in recent years [27]. Kaempferol has pharmacological activities such as antioxidant, anti-inflammation, and anti-tumor, and has been found through animal experiments to increase the levels of dopamine, NA, and 5-HT in the brain of depressed rats, thereby exerting antidepressant effects [28]. β-sitosterol is the main drug Incltains, study have found that β-sitosterol can manifest To increase the levels of 5-HT and NE in the brain of depressed mice depression-like behavior [29]. Consistent with the screened active ingredients in this study, we confirmed that quercetin and kaempferol may be one of the core effective materials of Radix Bupleuri-Paeoniae Radix Alba in treatment of PSD.
Further, our molecular docking experiments demonstrated quercetin and kaempferol interacted with key proteins, AKT1, IL-6 and IL-1β. Research has shown that IL-6 and IL-1β may play important roles in the occurrence and development of PSD, and inflammatory cytokines such as IL-6 and IL-1β can maintain high levels for several months after onset of stroke. This chronic immune inflammatory response can lead to mood changes associated with depression [30]. Chaihu-Shugan-San regulates the polarization of microglia through the JAK/STAT3-GSK3β/PTEN/Akt signaling pathway and subsequently inhibits neuroinflammation to ameliorate the symptoms of PSD. Meanwhile, Numerous studies have demonstrated that cytokine levels, such as IL-1β, IL-6, TNF-α, and IL-18, are signifcantly elevated in patients with PSD [31-33], suggesting that the presence of these cytokines in the brain may contribute to PSD development. Accordingly, the core proteins, AKT1, IL-6 and IL-1β, are instrumental in PSD, denoting that Radix Bupleuri-Paeoniae Radix Alba may exert anti-PSD activity by regulating these core proteins, and further in vitro experiments are needed to verify this hypothesis.
In addition, KEGG pathway analyses revealed that several signaling pathways were implicated in PSD treatment using Radix Bupleuri-Paeoniae Radix Alba, mainly including "lipids and atherosclerosis", "chemical carcinogenesis-receptor activation", "hepatitis B", "AGE-RAGE signaling pathway in diabetes complications" and "Kaposi sarcoma associated herpesvirus infection". According to a related report, atherosclerosis serves as one of the risk factors of PSD, and Dachaihu Tang containing "Radix Bupleuri-Paeoniae Radix Alba" can regulate blood fat, inhibit IL-1β expression and dampen the formation of atherosclerosis [34]. Moreover, emotions are closely related to the liver, with one-third of patients with hepatitis or cirrhosis experiencing depression. A key link between depression and liver disease seems to be the inflammatory process, in which increased microbiota and intestinal permeability play a crucial role [35]. While the findings of this study provide valuable insights into the potential mechanisms underlying the effects of Radix Bupleuri-Paeoniae Radix Alba in treating Post-Stroke Depression (PSD), it is essential to validate these results through rigorous animal experiments. Such studies are crucial for confirming the pharmacological effects of these compounds in vivo, assessing their bioavailability, and elucidating their mechanisms of action in a biological context.
5 Conclusion
Collectively, Radix Bupleuri-Paeoniae Radix Alba can mediate "lipids and atherosclerosis signaling pathway", "hepatitis B" and other signaling pathways, and act on core targets including AKT1, IL-6 and IL-1β via quercetin and kaempferol, thereby treating PSD. This study preliminarily predicts the mechanism of Radix Bupleuri-Paeoniae Radix Alba in PSD treatment, providing a theoretical basis for further research on its underlying mechanisms. Our results provide a new research base and biomarker for PSD treatment to improve treatment efficacy. Integration of these bioactive compounds into treatment options may improve patient outcomes by addressing the psychological and physiological aspects of rehabilitation, potentially improving the quality of life of the affected individuals.
Back Matter
Acknowledgments
Not applicable.
Conflicts of Interest
The authors declare no conflicts of interest.
Author Contributions
Y.F. and L.C. contributed to the conception and design of the paper. X.Z. and Z.Z. searched the literature and wrote the first draft of the manuscript. All the authors contributed to and approved the manuscript for submission.
Ethics Approval and Consent to Participate
The manuscript didn't involve any human or animal subjects, therefore no ethical approval was required for this article.
Funding
Project of Traditional Chinese Medicine Bureau of Guangdong Province (No. 20221330); Health System Research Project of Futian District (No. FTWS2022027).
Availability of Data and Materials
All data are available on request from the first author.
Supplementary Materials
Not applicable.
References
- Villa RF, Ferrari F, Moretti A. Post-stroke depression: Mechanisms and pharmacological treatment. Pharmacology & Therapeutics 2018; 184: 131-144.
- Ayerbe L, Ayis S, Wolfe CD, et al. Natural history, predictors and outcomes of depression after stroke: systematic review and meta-analysis. British Journal of Psychiatry 2013; 202(1): 14-21.
- Das J, Rajanikant GK. Post stroke depression: The sequelae of cerebral stroke. Neuroscience & Biobehavioral Reviews 2018; 90: 104-114.
- Vicentini JE, Weiler M, Almeida SRM, et al. Depression and anxiety symptoms are associated to disruption of default mode network in subacute ischemic stroke. Brain Imaging and Behavior 2017; 11(6): 1571-1580.
- Shi Y, Yang D, Zeng Y, et al. Risk Factors for Post-stroke Depression: A Meta-analysis. Frontiers in Aging Neuroscience 2017; 9: 218.
- Kootker JA, van Mierlo ML, Hendriks JC, et al. Risk Factors for Symptoms of Depression and Anxiety One Year Poststroke: A Longitudinal Study. Archives of Physical Medicine and Rehabilitation 2016; 97(6): 919-928.
- Shi Y, Xiang Y, Yang Y, et al. Depression after minor stroke: Prevalence and predictors. Journal of Psychosomatic Research 2015; 79(2): 143-147.
- Lin S, Luan X, He W, et al. Post-Stroke Depression and Estimated Glomerular Filtration Rate: A Prospective Stroke Cohort. Neuropsychiatric Disease and Treatment 2020; 16: 201-208.
- Choi-Kwon S, Han SW, Kwon SU, et al. Fluoxetine treatment in poststroke depression, emotional incontinence, and anger proneness: a double-blind, placebo-controlled study. Stroke 2006; 37(1): 156-161.
- Price A, Rayner L, Okon-Rocha E, et al. Antidepressants for the treatment of depression in neurological disorders: a systematic review and meta-analysis of randomised controlled trials. Journal of Neurology, Neurosurgery and Psychiatry 2011; 82(8): 914-923.
- Fang C, Zhang Z, Xu H, et al. Natural Products for the Treatment of Post-stroke Depression. Frontiers in Pharmacology 2022; 13: 918531.
- Zhao AM, Qiu WR, Mao LJ, et al. The efficacy and safety of Jiedu Tongluo granules for treating post-stroke depression with qi deficiency and blood stasis syndrome: study protocol for a randomized controlled trial. Trials 2018; 19(1): 275.
- Zhang Y, Yang Y, Feng Y, et al. The association between liver fibrosis and depression in patients after ischemic stroke. BMC Neurology 2023; 23(1): 50.
- Yuan M, Yuan J, Han Z. Analysis on the medication rules of traditional Chinese medicine in treat-ing poststroke depression based on traditional Chinese medicine inheri-tance support system. China Medical Herald 2021; 18: 159-162.
- Zhong Y, Li J, Zhu X, et al. A comprehensive review of bupleuri radix and its bioactive components: with a major focus on treating chronic liver diseases. Journal of Ethnopharmacology 2024; 330: 118244.
- Li J, Li X, Gao Y, et al. Clinical Application and Modern Pharmacological Research Progress of Radix Bupleurum and Paeoniae Alba. Journal of Liaoning University of Traditional Chinese Medicine 2019; 21(07): 8-12.
- Wang H, Zhou Q, Li B, et al. Chemical compositions and pharmacological effects of Chinese thorowax root: review. Jilin Journal of Chinese Medicine 2024; 44(01): 96-100.
- Tan YQ, Chen HW, Li J, et al. Efficacy, Chemical Constituents, and Pharmacological Actions of Radix Paeoniae Rubra and Radix Paeoniae Alba. Frontiers in Pharmacology 2020; 11: 1054.
- Wang Y, Gao SM, Li R, et al. Antidepressant-like effects of the Radix Bupleuri and Radix Paeoniae Alba drug pair. Neuroscience Letters 2016; 633: 14-20.
- Doncheva NT, Morris JH, Gorodkin J, et al. Cytoscape StringApp: Network Analysis and Visualization of Proteomics Data. Journal of Proteome Research 2019; 18(2): 623-632.
- Szklarczyk D, Gable AL, Lyon D, et al. STRING v11: protein-protein association networks with increased coverage, supporting functional discovery in genome-wide experimental datasets. Nucleic Acids Research 2019; 47(D1): D607-D613.
- Wang H, Mao S, Wang P, et al. Research advances in the pathogenesis and risk factors of post-stroke depression. Journal of Apoplexy and Nervous Diseases 2023; 40(09): 785-788.
- Shi Y, Yang D, Zeng Y, et al. Risk Factors for Post-stroke Depression: A Meta-analysis. Frontiers in Aging Neuroscience 2017; 9: 218.
- Du F, Fang L, Zhang C, et al. Clinical Effect of Yinao Jieyu Formula Combined with Acupuncture in the Treatment of Post Stroke Depression. Medical Innovation of China 2021; 18(09): 94-98.
- Wang C, Wu C, Yan Z, et al. Ameliorative effect of Xiaoyao-jieyu-san on post-stroke depression and its potential mechanisms. Journal of Natural Medicines 2019; 73(1): 76-84.
- Wang Y, Wang Y, Zou M, et al. Research progress on antidepressant effect and mechanism of quercetin and its glycoside derivatives. Chinese Herbal Medicine 2022; 53(05): 1548-1557.
- Ma N, Li Y, Fan J. Research Progress on Pharmacological Action of Quercetin. Journal of Liaoning University of Traditional Chinese Medicine 2018; 20(08): 221-224.
- Wang J, Xie J, Chen Y, et al. Antidepressant Effect of Kaempferol on Rat Complex Model of Depression and Breast Cancer. Chinese Journal of Modern Applied Pharmacy 2016; 33(03): 277-280.
- Zhao D, Zheng L, Qi L, et al. Structural Features and Potent Antidepressant Effects of Total Sterols and β-sitosterol Extracted from Sargassum horneri. Marine Drugs 2016; 14(7): 123.
- Xu Q, Qian X, Sun F, et al. Effects of crocin on inflammatory response and TLR4/MyD88/NF-κB signaling pathway in post-stroke depression rats. Chinese Journal of Immunology 2021; 37(02): 179-185.
- Kim JM, Kang HJ, Kim JW, et al. Associations of Tumor Necrosis Factor-α and Interleukin-1β Levels and Polymorphisms with Post-Stroke Depression. The American Journal of Geriatric Psychiatry 2017; 25(12): 1300-1308.
- Chen Y, Pu J, Liu Y, et al. Pro-infammatory cytokines are associated with the development of post-stroke depression in the acute stage of stroke: a meta-analysis. Topics in Stroke Rehabilitation 2020; 27(8): 620–629.
- Yang L, Zhang Z, Sun D, et al. The serum interleukin-18 is a potential marker for development of post-stroke depression. Neurological Research 2010; 32(4): 340-346.
- Song MY, Cho H, Lee S, et al. Daeshiho-tang Attenuates Atherosclerosis by Regulating Cholesterol Metabolism and Inducing M2 Macrophage Polarization. Life 2022; 12(2): 197.
- Kahl KG, Krüger T, Eckermann G, et al. Depressionen und Lebererkrankungen: Die Rolle von Mikrobiom und Inflammation [Major depression and liver disease: the role of microbiome and inflammation]. Fortschritte der Neurologie Psychiatrie 2019; 87(1): 12-21.